Joao Carlos AZEVEDO GONCALVES
Tuesday 26 March 2019 at 11.00am - IEMN, Amphithéâtre LCI, Campus cité scientifique, Villeneuve d'Ascq
Jury:
- Prof. Nathalie ROLLAND - IRCICA Lille (Chair)
- Pr. Dominique SCHREURS - KU Louvain (Rapporteur)
- Prof. Jean-Pierre RASKIN - UC Louvain (Rapporteur)
- Dr. Javier MATEOS - U Salamanca (Member)
- Dr. Jeremie TORRES - IES Montpellier (Member)
- Pr. François DANNEVILLE - IEMN Lille (Thesis supervisor)
- Prof. Christophe GAQUIERE - IEMN (Co-Director of thesis)
- Dr. Guillaume DUCOURNAU - IEMN Lille (Co-supervisor)
- Mr. Daniel GLORIA - ST Crolles (Industrial supervisor)
Introduction
Worldwide mobile data traffic has exploded in recent years, and is still growing. This is partly due to the fact that more and more connected objects such as telephones, smart TVs, intelligent sensors, surveillance cameras, coffee makers, etc. are becoming available to the general public. Coupled with this, new modes of data consumption such as social networking, 4K streaming and music on demand are tending to saturate the allocated communications channels. What's more, in the current context of territorial security, there is a growing need for fast, reliable systems to detect weapons, toxins or explosives for security purposes in airports, train stations or water distribution centres. Because of its properties, the millimetre frequency band defined between 30 GHz and 300 GHz is of great interest as a response to these problems. In fact, the millimetre frequency band could provide greater transmission bandwidth for telecommunications applications, resulting in an increase in available throughput. From an imaging point of view, the increase in operating frequency means greater precision and the ability to penetrate certain materials. Added to this, the attraction of the millimetre frequency range is all the greater because electrical dipoles interact strongly with radiation in this frequency range. Detecting chemical compounds using electronic systems means that spectroscopy can be carried out in real time, unlike chemical procedures, which can take several days.
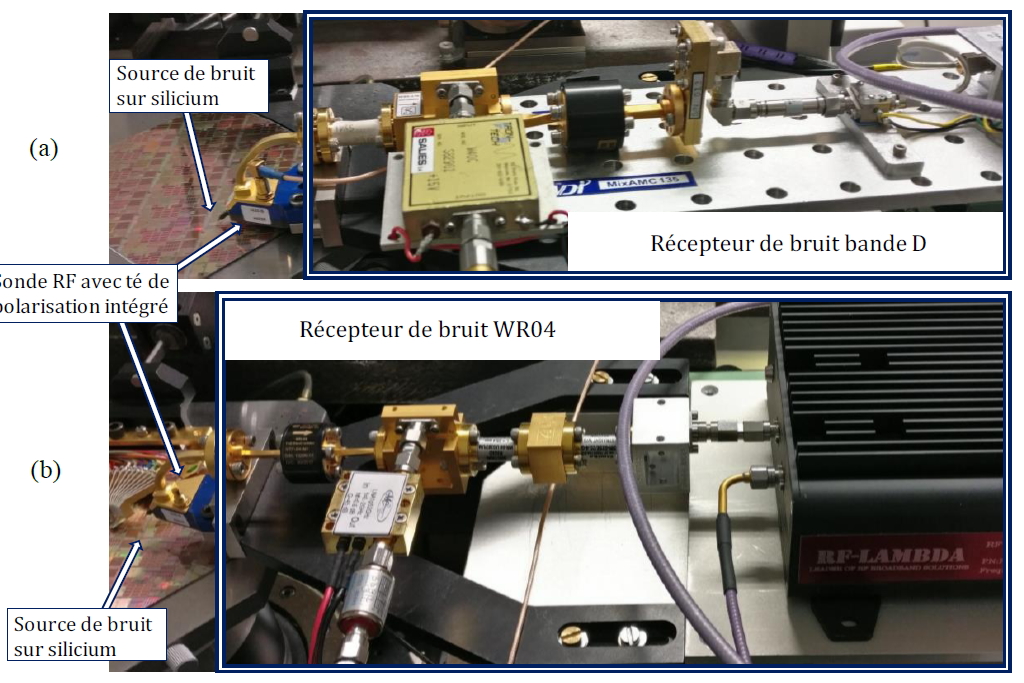
Photograph of the set-ups used for noise characterisation of silicon diodes (a) between 130 GHz and 170 GHz, (b) between 220 GHz and 230 GHz
Thanks to advances in silicon technology, it is now possible to design complex circuits in the millimetre frequency band above 100 GHz. The design of these systems is based on the use of accurate and reliable models of passive and active devices such as MOS or bipolar transistors. These models are themselves extracted and validated by high-frequency measurements up to 110 GHz. The high-frequency measurements carried out can be classified into three types of characterisation. Small signal characterisation is used to extract the frequency performance of the device. Large-signal characterisation is used to determine the device's non-linear behaviour. Finally, the aim of noise characterisation is to define the noise contribution of the device and extract its 4 noise parameters. In order to ensure the validity of these models, it is necessary to carry out measurements above 110 GHz. However, beyond this frequency, current characterisation benches and methods reach their limits. The IEMN laboratory and STMicroelectronics have been working together for a number of years, forming a joint laboratory, in order to address the problems associated with increasing the frequency of characterisation requirements. The aim of this thesis is to develop characterisation benches for measuring noise and power between 130 GHz and 320 GHz.